
By
Joshua Prentice
Since the very first space missions positioning, navigation, and timing (PNT) have been crucial for spaceborne applications. Traditionally, space vehicle PNT has been achieved through various combinations of ground stations, optical navigation, onboard high-precision clocks, inertial measurement units, and other methods. Only recently, however, has existing global navigation satellite systems (GNSS) been added to that list. GNSS constellations were designed to provide PNT for Earth-borne applications taking place on the ground, sea, or in the atmosphere. As such, those GNSS waveforms are primarily aimed toward the Earth, but there is a small amount of spill-over of the main lobe beyond the silhouette of Earth and into space. Additionally, the side lobes of most GNSS waveforms are also broadcast into space beyond Earth. Because these signals are visible from orbit, they can conceivably be used for the PNT of space vehicles. In terms of spaceborne navigation from GNSS constellations, there are generally two main orbital regions of concern. Altitudes between Earth and the GNSS altitude, known as being under the “canopy”, and altitudes above the GNSS canopy as shown below in Figure 1.

When orbiting the Earth underneath the GNSS canopy the receiver antenna must point “skyward” towards the GNSS constellations. This scenario is more closely related to traditional GNSS navigation, although satellites will rise and set more frequently. The full spectrum of these signals is available with the advantage of stronger signal strength compared to surface and low-atmospheric operations. In scenarios where the receiver vehicle is orbiting above the GNSS canopy, navigating based on GNSS constellations becomes much more difficult as the only available portions of the waveform are the main lobe spill-over and the side lobes. For simplification and to limit the scope of this tech brief, the primary area of concern will be space vehicles in geocentric orbits beneath the GNSS canopy.
When navigating from GNSS signals Doppler shift is always present no matter how close to the GNSS canopy the receiver is. However, when the navigating receiver is traveling at velocities necessary to maintain a stable orbit, the Doppler shift is much greater.

The Doppler shift change in frequency can be expressed as (Parker, 2017):

In equation (1) 𝑓₀ is the source carrier frequency, Δ𝑣 is the relative velocity of the space vehicles, and 𝑐₀ is the speed of light. This equation does not account for ionospheric and tropospheric effects encountered when GNSS signals pass through the Earth’s atmosphere. When considering equation (1) for multiple scenarios and orbital altitudes, the speed of light is a constant, and depending on the GNSS constellation being used so is the source carrier frequency. Thus, the biggest factor affecting Doppler shift is the relative velocity of the space vehicles. Because the satellites that make up GNSS constellations are held to very strict orbits with known orbital velocities and those orbits are maintained throughout the lifetime of the constellation, the determining factor of the relative velocity for any given mission is the orbital velocity of the receiver vehicle. It follows that the goal in computing a theoretical maximum Doppler shift a spaceborne receiver may encounter is to maximize the relative velocity between the receiver vehicle and the GNSS vehicle. A scenario that would accomplish this would be a receiver vehicle in very low earth orbit (VLEO) tracking GNSS signals.
Spaceborne missions taking place in LEO are a unique case of GNSS PNT due to the high relative velocity compared to the GNSS constellation vehicles while still being beneath the GNSS canopy. The dynamics of such a scenario are some of the highest that a receiver may experience during typical PNT operations. As such, the Doppler search space of receivers deployed in LEO must be much wider than needed for
ground, sea, and airborne missions.
One example of a very low earth orbit mission (VLEO) is the Gravity Field and Steady-State Ocean Circulation Explorer (GOCE). The GOCE mission required extremely precise orbit determination to carry out its scientific objective of mapping Earth’s gravity field to an accuracy of 1-2 cm. The GOCE space vehicle maintained an average orbital altitude of 255 km, placing the average orbital velocity around 8 ᵏᵐ⁄ₛ (European Space Agency, 2022). The GOCE mission tracked GPS signals to assist in orbit determination. GPS satellites orbit at an altitude of 20,200 km with an average orbital velocity of roughly 4 ᵏᵐ⁄ₛ (US Space Force, 2022).

Missions in VLEO have much shorter durations than other spaceborne missions due to the need for constant orbital maintenance maneuvers to counteract the atmospheric drag, and as such, it can be considered the lower limit of possible orbital altitudes. To estimate a maximum possible Doppler shift the worst possible case scenario would be the receiver satellite travelling in exactly the opposite direction (±180°) of the GNSS vehicle. While this is generally a very rare situation some space vehicles do travel in non-standard orbits, so it is possible.
Thus, the relative velocity of the space vehicles can be expressed as:

Where:
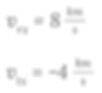
So that:

Note that all velocities are expressed as linear for simplification.
With an established relative velocity, the maximum estimated Doppler shift can be calculated using the following values:

Calculating the Doppler shift using the equation (1) results in:

With a worst-case-scenario Doppler shift of 63 kHz, it is imperative to ensure the receiver being placed into orbit can perform under such conditions.
Skydel Simulation Engine of the BroadSim product line is capable of simulating spaceborne scenarios, even under conditions where Doppler shift is maximized. One of the default vehicle profiles within Skydel is an Earth-orbiting spacecraft with highly customizable Keplerian elements to define the exact orbit thereceiver vehicle will experience. Should the default spacecraft profile not provide enough customization, Skydel can also be interfaced through hardware in the loop (HIL) where exact positions are pushed tothe simulator to simulate the specific trajectory of a receiver vehicle. Unlike some simulators where the Doppler shift will have to be either predetermined or manually added to the scenario, Skydel handles Doppler, ionospheric, and tropospheric effects automatically based on the scenario without requiring user input.

LEO and VLEO missions are becoming more and more popular especially in the fields of PNT, from both from a provider and user standpoint. To make sure those missions will have sufficient PNT capabilities advanced receivers will need to be used and new receivers will be developed to fill specific roles and advance current capabilities. While the Doppler shifts experienced by receivers on these missions will be high, robust testing to ensure mission success is capable using BroadSim simulation products powered by Skydel.
References
European Space Agency. (2022). GOCE Facts and Figures. Retrieved from https://www.esa.int/Applications/Observing_the_Earth/FutureEO/GOCE/Facts_and_figures
Parker, M. (2017). Digital Signal Processing 101. Elsevier Inc.
US Space Force. (2022). GPS: The Global Positioning System. Retrieved from https://www.gps.gov/systems/gps/space/#orbits