
By
Multiple Contributors
David Garigen (Orolia Defense & Security)
Alaiya Tuntemeke-Winter (Orolia Defense and Security)
Serge Grop, Stavros Melachroinos (Orolia)
The mRO-50 is Orolia’s new low SWaP-C miniaturized rubidium oscillator. It is an innovative new atomic clock designed to meet core requirements for military ground and mobile radio communications applications. While maintaining the same volume as a standard oven-controlled crystal oscillator (OCXO), it provides better holdover and higher stability with the lowest power consumption amongst other miniature atomic clocks (MACs) in the market. It can be a drop-in replacement for many applications.
The mRO-50 is lower cost than a Chip Scale Atomic Clock (CSAC), and due to its superior performance, small footprint, and low power consumption, it can be applied to many different applications. Namely, in telecommunications systems, it can improve the data throughput performance of networking waveforms, along with many non-networking waveforms. It can do this because its superior holdover and Allan deviation reduce the size of the guard bands, maximizing the volume of information that can be passed between radios.

Figure 1: Orolia's mRO-50
The transfer and acquisition of time in DoD and commercial applications from both GNSS and non-GNSS sources has become an important topic in recent decades with greater demands for precision. Many atomic clock products have been designed to strike a balance between performance and cost that allow these goals to be met. This paper describes how the use of the mRO-50 addresses problems that can be seen on ground or mobile platforms using the LTE waveforms as example. The importance of oscillator holdover and the benefits of synchronizing radios in the field are also discussed. The SWaP-C of the mRO-50 now opens the door for systems integrators to use atomic clocks in manpack and vehicular radio applications, replacing what has traditionally been a TCXO.
Modern radio communication requires that the transmitter and the receiver are tightly synchronized. This is to allow faster frequency hopping as well as the ability to network at high data rates largely due to a reduction in the size of the “guard bands”.
A great example of the benefits of time synchronization is LTE, a commercial networking waveform that is focused on high data throughput. The evolution of this waveform is indicative of the evolution of other, similar waveforms. Referencing table 1 below, one can see that the time/phase requirement of LTE is 1.5us, and the time/phase requirement of LTE-A is 500ns. In one generation, the waveform’s synchronization requirement got tighter by a factor of 3.

Table 1: LTE and LTE-A Requirements
For commercial radios, such as cell phones, a high precision sync can be achieved using commercial GPS.The problem with relying on GNSS signals is that, in military applications especially, they can become unavailable due to jamming and spoofing by adversaries- or by natural effects, such as “urban canyons”, or natural canyons, blocking a direct line of sight to satellites of interest. There are also instances in cities and in the field where devices can enter these areas for extended periods of time. In these GPS denied environments devices can no longer sync using GPS, forcing the device into holdover. When in holdover it is important for radios to maintain a precise clock. Though some waveforms have features to help keep them synced without GPS, these features limit data throughput because they use bandwidth for sending sync pulses instead of data. In many commercial devices the oscillator will quickly drift away from the actual time. Below we can see a comparison of holdover performance of common Oscillators using LTE and LTE-A as reference.
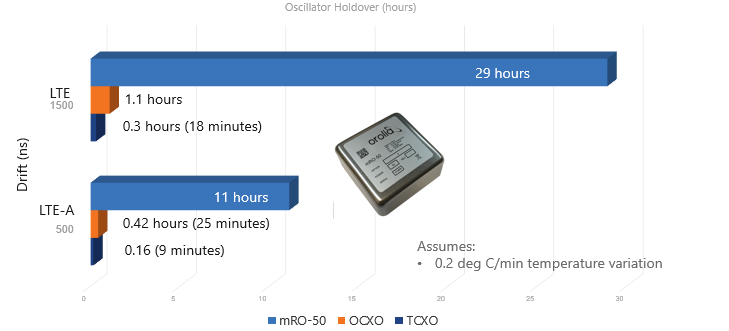
Figure 2: mRO, OCXO, and TCXO Comparison
When coming out of holdover, if a device is out of sync with its peers, it will need to synchronize before any meaningful communications can be performed. In this situation, many waveforms will need to enter a “cell search” mode or some equivalent form of peer synchronization prior to communicating. A cell search will waste time (it can take minutes for this sync to complete)- and it will force the user to perform multiple transmissions, increasing the opportunity of detection by an adversary. If, on the other hand, an atomic clock is used (such as an mRO-50)- and synchronization is maintained, the user can immediately start communicating- without the need for a cell search.
Guard Bands
The waveforms used in modern military radios operate by assigning time slots to users to allow them to simultaneously pass information (as observed by the operator). This technique in telecommunications is known as time division multiple access (TDMA). Thus, if there are many users in a group, such as an infantry platoon, then they can all transmit and receive on the same frequency, as the precise transmit and receive times are sliced and allocated amongst the users. Each time slot, however, requires some time at the start and at the end, known as “guard bands”, to prevent the radios from “talking over each other” and destroying the message. If the radios are all perfectly synchronized, then the guard bands can be reduced to zero- and data throughput is maximized.
In reality the radios are not well synchronized, and these guard bands can become significant, wasting valuable spectrum that could otherwise be used to pass more data. Thus, by improving the synchronization of the radios, the telecommunications system can maximize the data that can be passed over the air. The mRO-50 improves the timing synchronization, compared to a standard CSAC, by an order of magnitude due to its superior Allan deviation, bringing the system close to the ideal data throughput.
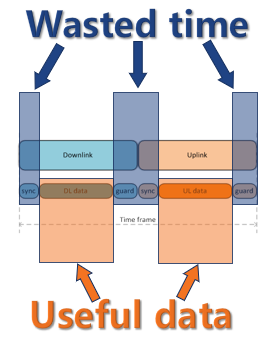
Figure 3: Why does sync matter?
Low SWaP-C, high precision atomic oscillators such as the mRO-50, also open the possibility for usage in UAV sensor payloads. These payloads often use GNSS to synchronize their clocks with other parts of the system. GPS outages can be problematic for both the UAV’s communications links and its inertial navigation systems (INS). The high stability of the atomic clock as the INS time base reduces the time integration error drift during extended GNSS outages, along with the telecommunications benefits already discussed. When the GNSS signal is disrupted, the UAV switches to the stable time base provided by the mRO-50 and can “coast” without a signal to maintain operations. The superior holdover time, offered by a mRO-50, allows the user to coast for a very long time. In fact, even when compared to a standard CSAC, the mRO-50 holdover time is more than 5x better in one day.
New possibilities for telecommunications systems and UAV sensor payloads have been realized with the advent of low SWaP-C atomic clocks, such as the mRO-50. Early adaptations of these advanced technologies keep our warfighters equipped with the best technologies and provide them with the tactical edge on the battlefield.
Conclusion
The importance of precision in the acquisition of timing from GNSS and non-GNSS sources has increased in recent decades. Many atomic clock products have been designed to strike a balance between performance and cost that allow this new degree of accuracy to be met. The mRO-50 is Orolia’s new low SWaP-C Miniaturized Rubidium Atomic Clock, specifically designed to meet core requirements foreground, and mobile radio communications applications in terms of weight, size, and performance. It will enable more advanced telecommunications systems and the resilience of UAVs and other GNSS dependent technologies. This technology can be a drop-in replacement for a simple upgrade, yielding superior performance in various applications.